Introduction
In biomechanical terms, the ankle and foot complex achieve stability and propulsion by coordinating the movements of 28 bones, forming 25 joints [
1]. The foot can bear substantial loads, adapt to different surfaces, and provide a rigid lever for push-off in gait, running, or jumping [
2]. In healthy adults, there is a significant correlation between hip abduction/adduction and rearfoot motion in the frontal and horizontal planes during gait [
3]. Therefore, any change in foot position can affect the position and movements of other parts of the lower limbs [
4]. The function of foot structures depends on the conditions that affect them [
5].
Musculoskeletal injuries related to foot and ankle are common among individuals engaged in occupational and sports activities [
6]. Many healthy people and those with musculoskeletal injuries are using orthoses. These devices serve the purpose of preventing and treating such injuries [
1,
2]. Several approaches have been developed to address or prevent these abnormalities, including biomechanically stabilizing and afferent stimulating measures [
7]. One biomechanical device utilized for postural adjustment is foot orthoses (FOs) [
8]. The orthotic elements in FOs can effectively reduce pressure beneath the metatarsal heads [
9,
10], induce changes in the geometry of the metatarsals and phalanges [
11, 12], adjust plantar aponeurosis strain [
13], promote or restrict tibial rotation [
14], and minimize rearfoot eversion/inversion [
14].
In recent years, new FOs have been developed that influence muscle activation by reinforcing sensory afferents and applying targeted pressure on specific foot regions using specific bars [
15]. German shoemakers initially created this type of orthosis to enhance the abnormal gait pattern in children with cerebral palsy, altering the lower extremity’s spastic tone by activating the foot’s plantar proprioceptors [
16]. This pressure can change the threshold of mechanoreceptors, thereby increasing neuronal stimulation. This way, the alpha motor neuron reflex inhibits, modulates, or activates the corresponding muscles through the muscle spindle afferent [
17, 18]. This condition can lead to active corrections in gait and postural control.
Different methods can significantly affect gait, postural control, and muscle activity. Biomechanical FOs focus on passively correcting the foot structure. Review studies confirm the effects of using these orthoses on gait, postural control, and muscle activity in different populations [
19-
21]. On the other hand, FOs with sensorimotor bars aim to modify mechanoreceptors’ afferent inputs. This systematic review aimed to determine whether FOs with sensorimotor bars change the gait, postural control, and muscle activity of healthy and musculoskeletal disorder individuals.
Materials and Methods
Study protocol
We used the PRISMA (the preferred reporting items for systematic reviews and meta-analyses) flowchart for the search and selection process [
22] and the Cochrane guidance for trusted systematic reviews for the data synthesis and presentation [
23].
Search methodology
The search strategy was defined based on systematic search rules. The principal investigator (Mahmood Bahramizadeh) selected the main keywords from free text and medical subject heading terms to create a search query based on PICO (patient/population, intervention, comparison and outcomes) items. One investigator (Hanieh Khaliliyan) conducted a computerized search in January 2024 using the PubMed, Scopus, and Web of Science databases.
Supplemental Appendix 1 shows the search query and obtained results from each database.
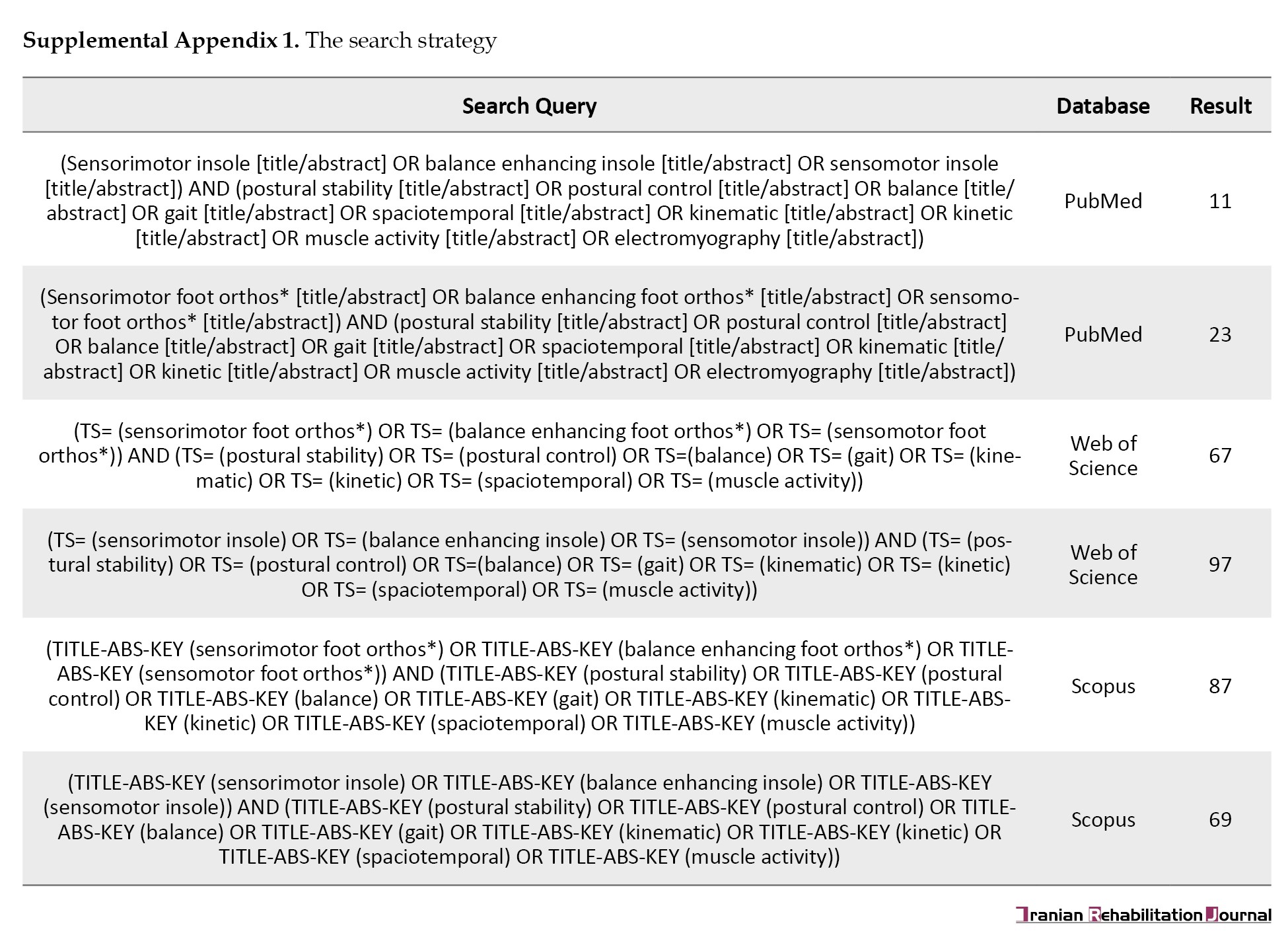
We uploaded the search findings to the online platform Covidence, which we used throughout the entire review procedure.
Study selection
All quantitative intervention studies evaluating any FOs with passive sensorimotor bars for healthy individuals and those with musculoskeletal disorders were eligible for inclusion, except for case reports, technical notes, and conference papers. The tools used to measure results could have been laboratory-based or clinical tests, but they should have focused on measuring outcomes related to gait, postural control, and muscle activity. We included peer-reviewed articles published in English. No restrictions were placed on the publication year to maximize the inclusion of studies. We excluded studies examining interventions involving FOs designed to improve the mechanical condition of lower limb joints, such as those with components like medial longitudinal arch support and heel cup. We also excluded FOs with active features like vibration systems. Firstly, duplicates were removed. Then, two independent investigators reviewed the “title” and “abstract” to determine if they met the inclusion criteria. Subsequently, two additional independent investigators assessed the full text, taking into account the inclusion criteria. During this phase, we used comments from two other investigators to resolve any disagreements.
Methodological quality
This review included studies based on the National Health and Medical Research Council classification at level 2 (randomized controlled trial) or level 3 (pseudorandomized and comparative controlled trial). We used the PEDro (the physiotherapy evidence database) scale to assess methodological quality. This scale consists of 11 items. We do not consider the first item regarding eligibility criteria for scoring purposes. We calculate the score on a scale from 0 to 10. Scores between 1 and 4 are considered poor, 5 to 6 fair, 7 to 8 good, and 9 to 10 excellent. Two independent investigators assess the methodological quality, and in case of disagreement, two other investigators resolve it.
Data extraction and synthesis
We extracted the data into a standardized table. This table consists of several columns based on suggestions from the cochrane handbook for systematic review of interventions [
30]. The column headings include author, publication year, study design, participants, intervention, protocol, assessment tools, outcomes, and findings. Also, Mean±SD, P, and outcomes statistics were collected in the form of Excel software, version 2019) using the standardized code book and were used to present the results. We performed a narrative synthesis of all data elements and discussed them in text form.
Results
Search results
The database search identified 354 articles, as shown in
Figure 1.
After removing duplicates and non-English studies, 229 studies underwent “title” and “abstract” screening based on inclusion criteria, resulting in 42 articles for full-text evaluation. Of the 42 articles, six included samples of people with stroke and Parkinson disease. Eight studies used mechanical bars instead of sensorimotor bars, while 11 studies evaluated satisfaction and performance using specific questionnaires. Finally, 17 studies met the inclusion criteria of this review study [
24-
40].
Methodological quality
Two investigators (Hanieh Khaliliyan and Mahmood Bahramizadeh) reviewed the 11 items of the PEDro checklist for each of the 17 included studies, resulting in 187 items. During the initial evaluation, they reached a consensus on 172 items, which accounts for 91% of the total. Cohen’s kappa inter-rater reliability assessment indicated a perfect consensus between the two investigators (k=0.946, 95% CI, 0.840%, 0.950%). With input from all investigators, we achieved a final agreement on all items (100%).
The Mean±SD score (6.58±1) indicates good study quality. Out of the 17 included studies, 16(94.11%) clearly stated the source and criteria of the included samples [
25-
40]. Eleven studies (64.70%) randomly allocated participants [
25-
29,
32,
33,
37-
40], whereas a researcher, unaware of the allocation procedure, included participants in 6 studies (35.29%) [
26,
31,
33,
38-
40]. The baseline data of study groups were similar in 15 studies (88.23%) [
25-
35,
37-
40]. In 6 studies (35.29%), the participants were blind [
26,
31-
33,
37,
38], and in 3 studies (17.64%), the therapist was blind [
38-
40]. All 17 studies (100%) obtained outcomes from more than 85% of the samples enrolled at the beginning of the studies, using intention-to-treat analysis [
24-
40]. Thirteen studies (76.47%) employed between-treatments statistical analysis [
25-
27,
29-
31,
33-
35,
37-
40], while 15 studies (88.23%) reported point and variability measures [
24-
38]. The National Health and Medical Research Council classification also determined the studies’ level.
Table 1 contains the details of the PEDro assessment and the level of studies.
Study characteristics
We summarized the results of the narrative synthesis of study data based on population, intervention, comparison, and outcome items. We present these results in textual and tabular formats (
Table 2).
Characteristics of population
The included studies evaluated a total of 541 samples. The mean sample size was 32 (range: 10-73). Among the participants, 319 were healthy, and 222 were patients with musculoskeletal disorders. Of the sample size, 215 were men, and 326 were women. The Mean±SD age of the participants was 24.45±15.84 years (range: 4-71). Their Mean±SD weight was 58.14±21.01 kg (range: 16-81), and mean height was 160.77±21.27 cm (range: 104-181).
Characteristics of sensorimotor bars
Sensorimotor FOs employed various bars to modify proprioceptive input. The main terms used in these studies to describe the type of bars were medial calcaneal, retrocapital, raised ridge, toe grip, proprioception, and peroneal pressure point bars. The medial calcaneal bar is an elevated wedge beneath the sustentaculum tali (5 studies) [
29,
34,
36-
38]. The retrocapital bar is an elevation with approximately 3 mm height situated posterior to the second to fifth metatarsal heads (6 studies) [
29,
30,
34,
36-
38]. The raised ridge bar is a plastic tube approximately 3 mm in diameter attached to the perimeter of the FOs with a 1 cm distance from the orthosis trim (4 studies) [
24, 25,
39, 40]. Five studies [
27, 28,
31-
33] positioned a raised toe-grip bar at the midpoint of the proximal phalanx, spanning the first to fifth toes. The proprioception bar is an evaluation area with a 3 mm wedge extending from the navicular to the hallux and sloped medial to lateral (1 study) [
35]. One study used a peroneal pressure point bar [
26]. This pressure point featured a concave shape on the plantar side to prevent the outer edge of the foot from rising mechanically and a convex shape on the dorsal side to apply pressure on the skin above the peroneus longus tendon about 8 mm distal to the inferior peroneal retinaculum [
26].
Comparing FOs with sensorimotor bars vs no FOs or other types of FOs
Three studies compared the sensorimotor FOs to prefabricated supportive FOs that included medial arch support and a heel cup [
35,
37, 38]. Two studies compared the effects of sensorimotor FOs to custom mold FOs [
39,
40]. Two other studies compared different sensorimotor FOs with various sensorimotor bars [
29,
34]. Five studies compared the sensorimotor FOs with no FOs [
28,
30,
32, 33,
36]. Some other studies used a flat FO as a placebo for the control condition [
25,
27,
31,
37, 38].
Gait outcomes
Kinematics
Sensorimotor bars increased foot external rotation compared to walking in shoes without FOs [
29,
34]. The implementation of the retrocapital and medial calcaneal bars significantly reduced internal rotation during the loading response (-18.3±28.1° vs -21.6±28.0°, P=0.009), mid stance (0.7±12.5° vs -2.0±14.9°, P=0.030), terminal stance (1.4±11.9° vs -2.3±14.5°, P=0.042), and terminal swing (-16.3±27.4° vs -19.0±26.4°, P=0.047) during in-toeing gait [
36].
Also, in healthy young adults, the medial calcaneal bar (MD±SD: -0.9±0.2, P=0.008), retrocapital bar (MD±SD: -2.0±0.2, P<0.001), and the combination of both bars (MD±SD: -2.0±0.2, P<0.001) all increased to the first peak of foot external rotation [
29]. Additionally, the medial calcaneal bar (MD±SD:1.0±0.1, P<0.001), retrocapital bar (2.0±0.1, P=0.04), and the combination of both bars (MD±SD:1.2±0.1, P<0.001) were associated with a decrease in the first peak of foot eversion. The first peak of foot dorsiflexion increased with the implementation of the medial calcaneal bar (MD±SD: -1.0±0.2, P<0.001) and the combination of both bars (MD±SD: -0.8±0.2, P=0.001), but no significant changes were observed with the retrocapital bar alone (MD±SD: -0.1±0.2, P=1.000) [
29,
34].
In young adults, the medial calcaneal bar significantly increased foot abduction across a majority of the stance phase (10%–90%, P<0.001), resulting in a peak value increase of 1.25° (P<0.001, r=0.4). Similarly, the retrocapital bar showed a rise in foot abduction for the entire stance phase, with a peak value increase of 2.19° (P<0.001, r=0.6) [
34]. Notably, the first peak of hip joint adduction exhibited no significant changes with the implementation of the medial calcaneal bar (MD±SD: -0.1±0.1, P=1), retrocapital bar (MD±SD: 0.1±0.1, P=1), or the combination of both bars (MD±SD: -0.1±0.1) [
29]. The use of a combination of the medial calcaneal and retrocapital bars during gait resulted in a significant reduction in the anteroposterior position of the hip (9%), shoulder (11%), and head (7%) in relation to the ankle joint in healthy young adults [
30].
Spatiotemporal parameters
The inclusion of the retrocapital and medial heel bars within FOs was associated with a significant improvement in walking speed (67.9 m/min versus 64.9 m/min, P<0.001) and stride length (500 mm vs 477 mm, P<0.001) in individuals with in-toeing gait. However, cadence did not significantly differ from individuals without FOs (137.6 steps/min vs 136.7 steps/min, P=0.89) [36]. In a study involving young adults, the utilization of toe grip bar FOs resulted in a greater step length (MD=2.81 cm, P=0.038), longer stance time (MD=0.03 s, P=0.001), and shorter swing time (MD=-0.02 s, P=0.003) compared to those using flat FOs [
31]. Additionally, in young adults, an increase in stride length was observed with the implementation of the medial calcaneal bar (MD±SD: -2.7±0.6, P<0.001) and the combination of the medial calcaneal bar and retrocapital bar (MD±SD: -2.9±0.6, P<0.001), while no significant change was noted with the retrocapital bar alone (MD±SD: -0.2±0.5, P=1) [
29].
Kinetics
When evaluating the impact of the proprioceptive bar, a significant reduction in maximum force was observed in the medial midfoot (MD±SD: 3.51±0.74). Conversely, an increase in plantar pressure was noted in the second and third rays (0.94±0.77 N/kg, 102.04±28.23 kPa) compared to wearing only shoes (1.12±0.88 N/kg vs 109.79±29.75 kPa) during gait in individuals withpes planovalgus (P<0.05) [
35]. Furthermore, the retrocapital bar led to a 15% decrease in foot plantar pressure compared to the control condition [
30].
Postural control outcomes
Clinical tests
In patients with ankle instability, the raised ridge bar significantly impacted various functional tests. It led to a substantial increase in errors during the single-leg stance test (2.96±2.1 vs 8.42±5.4, P<0.001, effect size: 2.18) but improved the reach distance in the single-leg hop test (110.8±18.2 vs 88.9±20.1, P<0.001, effect size=1.95) [
40]. Furthermore, this orthosis resulted in enhanced reach distances in the anteromedial (0.87±0.05 vs 0.94±0.07, P<0.001) and medial (0.89±0.04 vs 0.98±0.05, P<0.001) directions during the star excursion balance test compared to the custom mold FOs without this feature [
39]. Additionally, using the toe grip bar FO significantly improved the 25 m running time (6.72±0.53 vs 6.42±0.67, F=5.66, P=0.02) [
33]. However, this particular bar did not affect the results of the functional reach test in young adults [
27].
Center of pressure parameters
After a 12-week intervention using the peripheral raised ridge bar in healthy older adults, a significant decrease was noted in the mediolateral displacement of the center of pressure (COP) in relation to the lateral base of support limits during the single-limb support phase (6±2.9 vs 5.4±3.1 cm, F=7.92, P=0.035) [
25]. The raised ridge can effectively reduce COP excursion in the backward direction (P=0.003) in older adults, particularly within 1.5% to 6% of the base of support length [
24]. On the other hand, the retro capital bar did not significantly affect the COP’s position for each foot [
30].
During one-legged stance (48.32±15.57 vs 38.74±11.71, F=14.19, P<0.01) and two-legged stance (38.00±12.39 vs 32.97±11.52, F=6.84, P<0.01), the total length of the COP was significantly decreased when using the toe grip bar condition compared to the flat FOs [
28]. Conversely, the toe grip bar FOs did not significantly alter the total trajectory length and envelope area of COP in young adults [
27].
Muscle activity outcomes
Electromyography amplitude
The utilization of the retrocapital bar and medial calcaneal bar resulted in notable changes in muscle activity amongpes planovalgus patients. The amplitude of the peroneus longus muscle increased with a mean difference of -7.0 (95% CI, 0.7%, 13.3%; P=0.031) compared to a supportive FOs with a medial longitudinal arch [
37]. Conversely, the amplitude of the tibialis anterior muscle decreased with these bars, showing a mean difference of -9.19±20.12 compared to 0.00±3.90 without the bars, (95% CI, -15.97%, 2.41%; P=0.004) [
38]. Furthermore, during the loading response phase, the peroneus longus muscle exhibited an additional activity peak when using the peroneal pressure point FOs, peaking at 29.7% (±4.5%) of the stance phase in healthy young and older adults [
26].
Integrated electromyography
Individuals wearing FOs with a toe grip bar demonstrated significantly higher IEMG (integrated electromyogram) values for the tibialis anterior (21.71±8.97 vs 16.85±7.56, P=0.01) and the medial (28.34±13.33 vs 23.68±9.54, P=0.01) and lateral (28.74±15.21 vs 23.93±13.17, P=0.04) gastrocnemius muscles during the stance phase, as well as the tibialis anterior muscle (29.32±9.46 vs 22.20±7.68, P=0.001) during the swing phase of walking in young adults [
31, 32]. Additionally, a study involving healthy young and older adults found that the integration of electromyographic activity of the peroneus longus during the mid-stance phase was significantly higher with peroneal pressure point bars (18.1±11.3% MVCs) compared to control FOs (11.2±7.7% MVCs), with no substantial effects observed for the tibialis anterior or other gait phases [
26].
Discussion
Our review indicates that sensorimotor bars have positive effects on parameters related to gait (kinematics, kinetics, and spatiotemporal variables) [
29,
30,
34-
36], postural control (clinical tasks and COP displacement variables) [
27,
33,
39, 40], and electromyography [
24-
28,
30-
32,
37, 38]. These findings contribute to the increasing body of evidence that supports the significant role of plantar cutaneous mechanoreceptors in regulating different aspects of gait, postural control, and muscle activity.
Effects of sensorimotor bars on gait variables
Sensorimotor bars increased foot external rotation, dorsiflexion, and abduction while decreasing foot eversion [
29,
34,
36]. They also reduced the anteroposterior displacement of the hip, shoulder, and head [
30]. Furthermore, they observed significant improvements in walking speed [
36], stride length, stance time, swing time [
29,
31], and plantar pressure [
30,
35].
Positioning the calcaneus in a neutral position with the medial calcaneal bar during initial contact could reduce pronation of the subtalar joint and internal rotation dynamics of the leg [
36]. Participants with and without sensorimotor FOs showed similar cadence, which linked the speed increase to longer strides [
34]. This orthosis also encouraged a greater external rotation of the femur in relation to the pelvis towards the end of the swing phase, mirrored the internal rotation of the pelvis in relation to the femur. We might attribute the stride length augmentation to the pelvis rotating internally towards the walking direction [
29,
30].
The proprioceptive bar demonstrated efficacy (compared to control and prefabricated FOs conditions) in diminishing pressures on the medial midfoot, suggesting that stimulating the plantar surface can modify the foot’s biomechanics during gait [
35]. This outcome demonstrates the effectiveness of the FOs in reducing excessive pronation. According to Rothbart et al., this FOs generates tactile stimulation beneath the medial forefoot, which modifies sensory feedback to the brain and induces a postural adjustment in foot rotation [
41, 42].
The toe grip bar’s stimulation is believed to shift the foot’s pressure center forward, increasing stride length [
33]. Previous studies have suggested a relationship between forefoot pressure during the terminal stance and the push-off action [
43].
Effects of sensorimotor bars on postural control variables
Sensorimotor FOs improve the scores of clinical tests, including the single-leg hop test [
40], single-leg stance test [
40], star excursion balance test [
39], and 25-m running test [
33]. Furthermore, researchers found that these orthoses decreased the mediolateral [
25], backward [
24], and total length displacement of COP [
28]. The impact of stimulation may initially suggest an influence on functional tasks [
44]. We observed an average difference of around 10% in root-mean-square COP displacement during random platform movement between individuals who fell and those who did not. During continuous perturbation experiments, the stimulation restricted the COP’s movement towards the anterior or lateral [
45].
Proprioceptive sensors in the joints and muscles may provide information that explains the different effects of skin sensation, depending on the direction and type of response [
24]. When the feet are stationary, toe sensors may provide accurate measurements of anterior and lateral shifts in the center of mass while relying more on the skin mechanoreceptors to control the center of mass movement (and prevent stepping) in the backward direction [
45]. In responses that involve stepping, the foot typically makes contact near the front when stepping lateral or backward, so toe proprioception could help identify and control the landing [
46]. Our review results indicate that sensory stimulation decreases mediolateral and total length displacement of COP. However, we have observed that applying hypothermic anesthesia to the foot plantar surface elevates these parameters [
47, 48].
Effects of sensorimotor bars on muscle activity
Sensorimotor bars increase peroneus longus [
26,
37] and gastrocnemius electromyography [
32], but the results are conflicting regarding the tibialis anterior muscle [
26,
31, 32,
38]. An agonist muscle contraction triggers reciprocal inhibition, also known as automatic antagonist alpha motor neuron suppression. Moreover, the study found that sensory stimulation enhances antagonist reciprocal inhibition. Hence, the study proposes that heightened sensory performance enhances antagonist reciprocal inhibition, enhancing muscle activity [
49].
Pes plano valgus feet showed reduced peroneus longus activity during initial contact and mid-stance. Conversely, the tibialis anterior and posterior exhibit heightened muscle activity during mid-stance. Researchers attributed this shift to excessive strain on the medial longitudinal arch, which prompted a compensatory load adjustment [
37,
38]. Variances in baseline muscle activity between healthy individuals and those withpes planovalgus may elucidate the diverse outcomes regarding the impact of sensorimotor FOs on tibialis anterior muscle engagement.
Conclusion
Previous research has shown that using FOs with sensorimotor bars can improve motor function. These orthoses have the potential to enhance gait kinematics by increasing foot external rotation, dorsiflexion, and abduction while simultaneously decreasing foot eversion. Additionally, they can reduce the anteroposterior displacement of the hip, shoulder, and head. Furthermore, we have observed significant improvements in spatiotemporal parameters like walking speed, stride length, stance time, swing time, and kinetic parameters like plantar pressure. These orthoses can also impact clinical tasks and mitigate COP movements, thus aiding postural control. Lastly, they can potentially improve the activity of the peroneus longus and gastrocnemius muscles.
Study limitations
Previous studies have utilized various sensorimotor bars to stimulate different sensory points on the foot plantar surface. However, the specific mechanism of each function is still unknown. Furthermore, future studies need to consider excluding factors such as learning and placebo effects of the FOs with sensorimotor bars. Note that all the studies conducted so far have occurred in controlled environments. So, the results of using these orthotic devices in real-life situations where visual cues are limited because of poor vision, poor lighting, or cognitive tasks that affect visual-spatial perception have not been shared yet.
Ethical Considerations
Compliance with ethical guidelines
There were no ethical considerations to be considered in this research.
Funding
This research did not receive any grant from funding agencies in the public, commercial, or non-profit sectors.
Authors' contributions
Conceptualization, methodology and supervision: Mahmood Bahramizadeh; Investigation, data collection and data analysis: Hanieh Khaliliyan, and Mahmood Bahramizadeh; Writing the original draft: Hanieh Khaliliyan; Review and editing: Alireza Khaghani, Shahla Mohajeri, Francesco Chirico, Kavita Batra, Lukasz Szarpak, Majid Ansari, Aanuoluwapo Afolabi, Olayinka Ilesanmi, Gabriella Nucera, Hicham Khabbache, Farhad Ghaffari, Arash Sharafatvaziri, Mohammad Taghi Karimi.
Conflict of interest
The authors declared no conflict of interest.
References
- Levangie PK, Norkin CC. Joint structure and function: A comprehensive analysis. Philadelphia: FA Davis; 2011. [Link]
- Wong DW, Ni M, Wang Y, Zhang M. Biomechanics of foot and ankle. In: Cheng CK, Woo SLY, editors. Frontiers in orthopaedic biomechanics. Singapore: Springer; 2020. [DOI:10.1007/978-981-15-3159-0_10]
- Koshino Y, Yamanaka M, Ezawa Y, Okunuki T, Ishida T, Samukawa M, et al. Coupling motion between rearfoot and hip and knee joints during walking and single-leg landing. Journal of Electromyography and Kinesiology. 2017; 37:75-83. [DOI:10.1016/j.jelekin.2017.09.004]
- Cote KP, Brunet ME, Gansneder BM, Shultz SJ. Effects of pronated and supinated foot postures on static and dynamic postural stability. Journal of Athletic Training. 2005; 40(1):41-6. [PMID]
- Bagherzadeh Cham M, Mohseni-Bandpei MA, Bahramizadeh M, Forogh B, Kalbasi S, Biglarian A. Effects of vibro-medical insoles with and without vibrations on balance control in diabetic patients with mild-to-moderate peripheral neuropathy. Journal of Biomechanics. 2020; 103:109656. [DOI:10.1016/j.jbiomech.2020.109656] [PMID]
- Ait Ali D, Oukhouya K, Aziz A, Bouhali H, El Khiat A, El Koutbi M, et al. Prevalence of musculoskeletal disorders among healthcare professionals: A hospital-based study. Advances in Medicine, Psychology, and Public Health. 2024; 1(1):12-25. [Link]
- Kiaghadi A, Bahramizadeh M, Hadadi M. Effect of textured and prefabricated insole use with medical or sports shoes on dynamic postural control in elderly people. Topics in Geriatric Rehabilitation. 2020; 36(1):55-9. [DOI:10.1097/TGR.0000000000000255]
- Stacoff A, Kramers-de Quervain I, Dettwyler M, Wolf P, List R, Ukelo T, et al. Biomechanical effects of foot orthoses during walking. The Foot. 2007; 17(3):143-53. [DOI:10.1016/j.foot.2007.02.004]
- Hastings MK, Mueller MJ, Pilgram TK, Lott DJ, Commean PK, Johnson JE. Effect of metatarsal pad placement on plantar pressure in people with diabetes mellitus and peripheral neuropathy. Foot & Ankle International. 2007; 28(1):84-8. [DOI:10.3113/FAI.2007.0015]
- Lee PY, Landorf KB, Bonanno DR, Menz HB. Comparison of the pressure-relieving properties of various types of forefoot pads in older people with forefoot pain. Journal of Foot and Ankle Research. 2014; 7(1):1-18. [DOI:10.1186/1757-1146-7-18]
- Michaud TC, Nawoczenski DA. The influence of two different types of foot orthoses on first metatarsophalangeal joint kinematics during gait in a single subject. Journal of Manipulative and Physiological Therapeutics. 2006; 29(1):60-5. [DOI:10.1016/j.jmpt.2005.11.009] [PMID]
- Ward M, Johnson C, Klein J, Farber JM, Nolin W, Peterson MJ, et al. Orthotics and assistive devices. Pediatric Rehabilitation Principles and Practice. 2021; 269-304. [Link]
- Sinclair J, Isherwood J, Taylor PJ. The effects of orthotic intervention on multisegment foot kinematics and plantar fascia strain in recreational runners. Journal of Applied Biomechanics. 2015; 31(1):28-34. [DOI:10.1123/jab.2014-0086] [PMID]
- Eslami M, Begon M, Hinse S, Sadeghi H, Popov P, Allard P. Effect of foot orthoses on magnitude and timing of rearfoot and tibial motions, ground reaction force and knee moment during running. Journal of Science and Medicine in Sport. 2009; 12(6):679-84. [DOI:10.1016/j.jsams.2008.05.001] [PMID]
- Baur H, Hirschmüller A, Jahn M, Müller S, Mayer F. Therapeutic efficiency and biomechanical effects of sport insoles in female runners. Journal of Foot and Ankle Research. 2008; 1 (Suppl S1): O14-O. [DOI:10.1186/1757-1146-1-S1-O14]
- Hafkemeyer U, Poppenborg D, Drerup B, Möller M, Wetz HH. Improvements of gait in paraplegic patients using proprioceptive insoles. Münster: Universitätsklinikum Münster; 2003. [Link]
- Macefield VG, Knellwolf TP. Functional properties of human muscle spindles. Journal of Neurophysiology. 2018; 120(2):452-67. [DOI:10.1152/jn.00071.2018]
- Takakusaki K. Functional neuroanatomy for posture and gait control. Journal of Movement Disorders. 2017; 10(1):1-17.[DOI:10.14802/jmd.16062] [PMID]
- Khaliliyan H, Sharafatvaziri A, Safaeepour Z, Bahramizadeh M. Gait and muscle activity measures after biomechanical device therapy in subjects with ankle instability: A systematic review. The Foot. 2024; 59:102083. [DOI:10.1016/j.foot.2024.102083] [PMID]
- Bahramizadeh M, Khaliliyan H. Efficacy of different types of foot orthoses on postural control in subjects with lateral ankle sprain: A systematic review. Iranian Rehabilitation Journal. 2022; 20(3):287-96. [DOI:10.32598/irj.20.3.1719.1]
- Aboutorabi A, Bahramizadeh M, Arazpour M, Fadayevatan R, Farahmand F, Curran S, et al. A systematic review of the effect of foot orthoses and shoe characteristics on balance in healthy older subjects. Prosthetics and Orthotics International. 2016; 40(2):170-81. [DOI:10.1177/0309364615588342] [PMID]
- Liberati A, Altman DG, Tetzlaff J, Mulrow C, Gøtzsche PC, Ioannidis JP, et al. The PRISMA statement for reporting systematic reviews and meta-analyses of studies that evaluate health care interventions: Explanation and elaboration. Plos Medicine. 2009; 6(7):e1000100. [DOI:10.1371/journal.pmed.1000100] [PMID]
- Cumpston M, Li T, Page MJ, Chandler J, Welch VA, Higgins JP, et al. Updated guidance for trusted systematic reviews: A new edition of the Cochrane Handbook for Systematic Reviews of Interventions. The Cochrane Database of Systematic Reviews. 2019; 10(10):ED000142. [DOI:10.1002/14651858.ED000142] [PMID]
- Maki BE, Perry SD, Norrie RG, McIlroy WE. Effect of facilitation of sensation from plantar foot-surface boundaries on postural stabilization in young and older adults. Journals of Gerontology Series A: Biomedical Sciences and Medical Sciences. 1999; 54(6):M281-7. [DOI:10.1093/gerona/54.6.M281] [PMID]
- Perry SD, Radtke A, McIlroy WE, Fernie GR, Maki BE. Efficacy and effectiveness of a balance-enhancing insole. The Journals of Gerontology Series A: Biological Sciences and Medical Sciences. 2008; 63(6):595-602. [DOI:10.1093/gerona/63.6.595] [PMID]
- Ludwig O, Kelm J, Fröhlich M. The influence of insoles with a peroneal pressure point on the electromyographic activity of tibialis anterior and peroneus longus during gait. Journal of Foot and Ankle Research. 2016; 9(1):33. [DOI:10.1186/s13047-016-0162-5] [PMID]
- Nakano H, Murata S, Abiko T, Sakamoto M, Matsuo D, Kawaguchi M, et al. Effect of insoles with a toe-grip bar on toe function and standing balance in healthy young women: A randomized controlled trial. Rehabilitation Research and practice. 2017; 2017:2941095. [DOI:10.1155/2017/2941095] [PMID]
- Nakano H, Murata S, Abiko T, Sakamoto M, Matsuo D, Kawaguchi M, et al. Effect of insoles with a toe-grip bar on toe-grip strength and body sway in middle-aged and elderly women. Topics in Geriatric Rehabilitation. 2019; 35(2):125-8. [DOI:10.1097/TGR.0000000000000221]
- Laštovička O, Klein T, Abrantes J, Janura M. Immediate effect of individual bars of insoles and their combination on gait parameters in asymptomatic healthy adults. Somatosensory & Motor Research. 2020; 37(2):125-31. [PMID]
- Vermand S, Duc S, Janin M, Ferrari FJ, Vermand M, Joly P. The influence of insole with metatarsal retro-capital on posture, plantar pressure and body segments positions in runners. International Journal of Clinical Medicine. 2019; 10(05):326-35. [DOI:10.4236/ijcm.2019.105025]
- Abiko T, Murata S, Kai Y, Nakano H, Matsuo D, Kawaguchi M. Differences in electromyographic activities and spatiotemporal gait parameters between general and developed insoles with a toe-grip bar. BioMed Research International. 2020; 2020. [DOI:10.1155/2020/6690343]
- Nakano H, Murata S, Kai Y, Abiko T, Matsuo D, Kawaguchi M. The effect of wearing insoles with a toe-grip bar on occupational leg swelling and lower limb muscle activity: A randomized cross-over study. Journal of Occupational Health. 2020; 62(1):e12193. [DOI:10.1002/1348-9585.12193] [PMID]
- Nakano H, Murata S, Abiko T, Mitsumaru N, Kubo A, Hachiya M, et al. Effects of long-term use of insoles with a toe-grip bar on the balance, walking, and running of preschool children: A randomized controlled trial. BioMed Research International. 2020; 2020:1940954. [DOI:10.1155/2020/1940954] [PMID]
- Klein T, Lastovicka O, Janura M, Svoboda Z, Chapman GJ, Richards J. The immediate effects of sensorimotor foot orthoses on foot kinematics in healthy adults. Gait & Posture. 2021; 84:93-101. [DOI:10.1016/j.gaitpost.2020.11.022] [PMID]
- Aminian G, Safaeepour Z, Farhoodi M, Pezeshk AF, Saeedi H, Majddoleslam B. The effect of prefabricated and proprioceptive foot orthoses on plantar pressure distribution in patients with flexible flatfoot during walking. Prosthetics and Orthotics International. 2013; 37(3):227-32. [DOI:10.1177/0309364612461167] [PMID]
- Mabuchi A, Kitoh H, Inoue M, Hayashi M, Ishiguro N, Suzuki N. The biomechanical effect of the sensomotor insole on a pediatric intoeing gait. International Scholarly Research Notices. 2012; 2012:396718. [DOI:10.5402/2012/396718] [PMID]
- Schmitt AP, Liebau KH, Hamm A, Hacke C, Mittelmeier W, Schulze C. Comparison of the influence of supportive and sensorimotor insoles in the muscle activity of tibialis anterior and peroneus longus in combat boots. The Foot. 2022; 52:101910. [DOI:10.1016/j.foot.2022.101910] [PMID]
- Liebau KH, Schmitt AP, Fröhlich S, Bünzen C, Mittelmeier W, Schulze C. Comparison of the influence of supportive and sensorimotor insoles on flat feet in children-a double-blind, prospective, randomized, controlled trial. Ortopedia, Traumatologia, Rehabilitacja. 2023; 25(4):195-206. [DOI:10.5604/01.3001.0053.9346] [PMID]
- Khaliliyan H, Bahramizadeh M, Vahab Kashani R, Vahedi M. Effects of the custom mold with a raised ridge around the perimeter foot orthoses on dynamic postural control in chronic ankle instability. Iranian Rehabilitation Journal. 2023; 21(3):451-60. [DOI:10.32598/irj.21.3.1719.2]
- Khaliliyan H, Bahramizadeh M, Kashani RV, Vahedi M. Effects of custom mold with peripheral textured surface foot orthosis on balance and physical function in subjects with chronic ankle instability. Advances in Medicine, Psychology, and Public Health. 2024; 1(2):74-81. [Link]
- Rothbart BA. Proprioceptive insoles. From a podiatric point of view. Health Healing Wisdom. 2005; 29(11). [Link]
- Rothbart BA. Medial column foot systems: An innovative tool for improving posture. Journal of Bodywork and Movement Therapies. 2002; 6:37-46. [Link]
- Li B, Zhang X. The dynamic characteristics of the center of pressure for toe-out gait: Implications for footwear design. Journal of Leather Science and Engineering. 2022; 4(1):12. [DOI:10.1186/s42825-022-00088-1]
- Khaliliyan H, Ansari M, Bahramizadeh M, Sharafatvaziri A, Ghaffari F, Karimi M. Postural stability efficacy of textured shoe inserts for ankle instability patients: A systematic review. Advances in Medicine, Psychology, and Public Health. 2025; 2(1):8-18. [Link]
- Zaback M, Adkin AL, Chua R, Inglis JT, Carpenter MG. Facilitation and habituation of cortical and subcortical control of standing balance following repeated exposure to a height-related postural threat. Neuroscience. 2022; 487:8-25. [DOI:10.1016/j.neuroscience.2022.01.012]
- Sotirakis H, Patikas D, Stergiou N, Hatzitaki V. Swaying to the complex motion of a visual target affects postural sway variability. Gait & Posture. 2020; 77:125-31. [DOI:10.1016/j.gaitpost.2020.01.026] [PMID]
- Asai H, Fujiwara K, Tachino K. Limiting factor for movable range of the centre of foot pressure in backward direction. In: Taguchi K, Igarashi M, Mori S, editors. Vestibularand Neural Front. Tokyo: Elsevier; 1994. [Link]
- Perry SD, McIlroy WE, Maki BE. The role of plantar cutaneous mechanoreceptors in the control of compensatory stepping reactions evoked by unpredictable, multi-directional perturbation. Brain Research. 2000; 877(2):401-6. [DOI: 10.1016/S0006-8993(00)02712-8]
- García González L. Exploration of peripheral electrical stimulation adapted as a modulation tool for reciprocal inhibition through the activation of afferent fibers during gait (MSc thesis). Barcelona: Higher Technical School of Industrial Engineering; 2023. [Link]